The African Wild Dog
Muscles & Skeleton
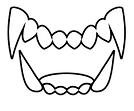
TEETH
The African wild dog’s has a short and wide muzzle, which allows them to tight grasp and hold on to their prey. Their premolars have evolved to be more blade-like, or carnassial, meaning they're adapted for shearing flesh. This makes them well-suited to taking down prey. An added benefit of having razor-sharp teeth is it decreases consumption time at the kill. Painted wolves are slightly built – the faster they can eat their meal and get out of there, the less likely they will encounter other bigger predators like lion, leopard, and hyena. Their large post-carnassial molars are strong enough to break up large bones.
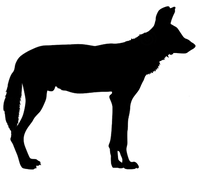
Muscles
African wild dogs have a vestigial first digit and muscular adaptations for their cursorial lifestyle. Muscles, ligaments, and bones of the forearm are adapted to provide stability to the wrist and elbow, which assists in jumping and with long-distance endurance running to target exhaustion in their prey. Their legs are long and muscular which promote their fast running speeds, reaching highs of 44mph (for reference, the cheetah runs at an average of 50-80 mph and the domestic dog runs at an average of 30 mph).
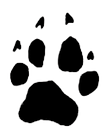
four toes
The African Wild Dog has four toes, unlike other dogs (i.e. domestic) that typically have a fifth toe, the dewclaw. This species evolved to only have four due to their nature of running. These wild dogs are agile hunters that travel over long distances tracking their prey, known as "cursorial animals." They are distinguished for their elongated limbs, shortened digits, and reduced number of toes.

The dental formula is incisors (I) 3/3, canines (C) 1/1, premolars (PM) 3/4, and molars (M)3/3= 42 total teeth, where the last mandibular molar is vestigial and typically not featured. This formation allows for them to tear through and devour large amounts of meat and bone with speed.

Figure.
African Wild Dog (Lycaon pictus) skull (left) compared with the skull of the Wolf (Canus lupus) (right).
When compared, you can see the wild dog's shorter and wider muzzle along with fewer molars than the wolf.
Skull and Dental
The African wild dog's skull is equipped with strong muscles and modified upper incises (i.e. sharp teeth used to bite into food), which provide for their extremely strong bite. Their sharp canines are used in hunts to grip onto prey and bring them down. The carnassial (i.e. paired upper and lower teeth) pass by each other in a motion that slices the flesh and meat of their prey. This shearing capacity is a huge benefit for the dogs, where they can consume their prey in record times. An added benefit for them is that their teeth are capable of sharpening themselves, so these hunters are almost always prepared. Their large, sharp premolars are relative to their body mass.
A study was conducted to estimate bite force across a variety of canids of hypercarnivore predators that tend to hunt prey larger than themselves. This study found the bite force at the canines (in newtons/kilogram of body weight) in African wild dogs to be 142 N/kg. Comparatively, the extinct dire wolf was among the greatest at 163 N/kg, where the gray wolf came in at 136 N/kg, the dhole at 112 N/kg, and the dingo at 108 N/kg. The bite force at the carnassial teeth revealed similar data to the canines. Conclusively, a predator's largest prey size is dependent upon its biomechanical limits, where the African wild dog shows little limitations.
Most canids, including the gray wolf, the talonid retains two main cusps and has a crushing function. However, in the African wild dog, the lingual entoconid (Ent) has been lost and the labial hypoconid (Hyp) is converted into a small blade, which fits into a basin in the first upper molar (figure). ​
The modified talonid of the lower carnassial is referred to as the trenchant heel (figure).
The molars immediately behind the carnassials are large, especially in the upper jaw, while the last molars are smaller. The molars are used to crush bones.

Figure.
Dentition of the African wild dog (Lycaon pictus).
(A) Lateral view of skull. Original image width = 24.5 cm.
(B) Occlusal view of upper dentition (left) and lower dentition (right). Arrows indicate trenchant heels on lower carnassials. Original image width = 31.6 cm.
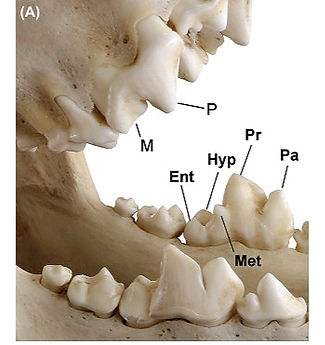
Fig. Carnassial teeth.
(A) Permanent carnassials of the gray wolf (Canis lupus). Cusps on the lower-left carnassial are labeled.
Upper carnassial: M, metacone; P, paracone. Lower carnassial: Ent, entoconid; Hyp, hypoconid; Met, metaconid; Pa, paraconid; Pr, protoconid.
Skeletal System

Figure.
The African Wild Dog's skeleton is more closely related to a raccoon than a dog. They are also thin and muscular. 
Notably, the African wild dog's skeleton is actually more closely related to a raccoon than a dog. However, reproductive anatomy is similar to the domestic dogs in both males and females, where females typically have between 12–14 mammae (i.e. milk-secreting organs). Their body’s skeletal build is thin and muscular, where their long legs help with endurance and speed (up to ~44mph).  ​
All wolf-like Canids have a chromosome number of 2n=78. Canis species can interbreed or hybridize to produce fertile offspring, however the wild dogs are specific in their makeup that they would be infertile with other canids.
Like other dogs, their shoulder blades are not directly connected to the rest of their skeletal system and are only attached by muscles, ligaments, and tendons.
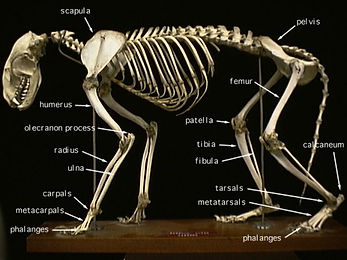
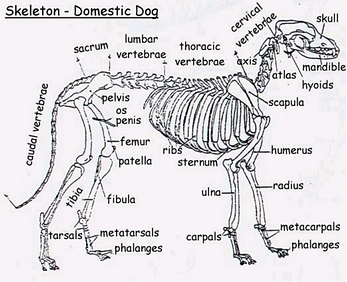
Figure.
Skeletal system of the raccoon (top) compared to the domestic dog (bottom). Note that the drawn figure (left) is more similar to the raccoon than that of the domesticated dog.
Muscles
African wild dogs have a vestigial first digit prevalent under the skin. This vestigial dewclaw hasn't formed any bone but rather has muscles attached. Due to adaptations, the muscles in the African wild dog have reorganized and repurposed themselves to fit their lifestyle, compared to other canid species.
The muscular adaptations of the vestigial first digit, or metacarpal I, function to support the wrist, where they would originally be moving the first digit. Their wrists have strong spring ligaments that allow for agility and passive action when shifting locomotor forces.
In comparison to other canids, the wild dogs have a reduced forelimb muscles, which rotate the limb, but on the other hand, muscles that store elastic energy have expanded.
​
The adaptations of the forelimb indicate the L. pictus are built for endurance running. The vestigial first digit and wrist adaptations allow for strong spring ligaments in passive actions. Further, these structural modifications allow for greater energy storage in their muscles and an increase in stability.
Figure.
Illustration of the muscles and bones of the African wild Figure 8: Medial view of the right antebrachium in L. pictus, including mm. biceps brachii and brachioradialis.
​
Illustration of the muscles and bones of the African wild dog forearm. The bone labeled "metacarpal 1" is the vestigial first digit. Muscles of the forearm are adapted to provide stability to the wrist and elbow, which assists with endurance running.
Illustrations drawn by Brent Adrian of Midwestern University.
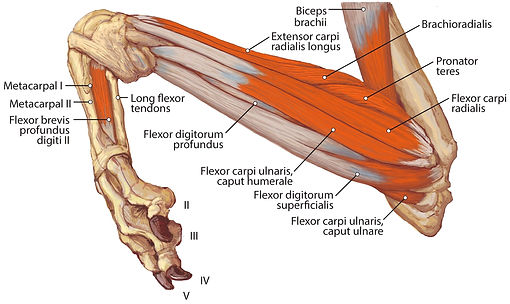
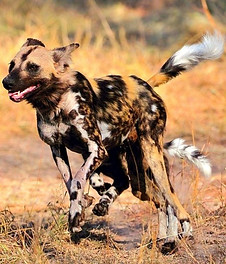

Figure
Palmar view of manus muscles in L. pictus (right side): (A) superficial view; (B) deep view.

The dogs have a similar muscular system to other Canids, with respect to their unique evolutionary adaptations to being cursorial hunters. The muscle system performs three functions that help maintain homeostasis: movement, support, and heat production.
​
Canids, like the African wild dog, build for endurance rather than speed. Accordingly, their muscle structure and functions have adapted to their lifestyle. These adaptations aid in running, jumping, stalking, and engaging with others.
​
Muscles are controlled either voluntarily or involuntarily. Voluntary muscles are typically controlled by thought, where there is intention when utilizing them (e.g. legs, tail, vocals). On the other hand, involuntary muscles cannot be controlled by thought and are regulated by the body's systems (e.g. heart, digestion). Involuntary muscles are typically smooth or non-striated muscles.
There are three types of muscle tissue:
​
-
Smooth muscle:
-
found in internal organs
-
(i.e. intestines, stomach, and bladder)
-
-
are involuntary muscles
-
are under the control of the autonomic nervous system
-
-
Skeletal muscle:
-
are striated muscles
-
are voluntary muscles
-
predominately attach to portions of the skeleton
-
involved in activities like locomotion, eating, tail wagging and eye movement
-
-
Cardiac muscle:
-
found in the heart
-
are involuntary muscles
-
unique in that it is a striated muscle, like skeletal muscle, but is not under voluntary control.
-
Muscular System

Strong, elastic connective tissue tendons help attach skeletal muscle to the bones. When muscles contract, they pull on the tendons, which then pull on the bones and allow the limbs to move. During contraction, myosin bind to actin and pull actin inward which demands energy from adenosine triphosphate (ATP) cells. At binding sites, enzymes hydrolyze ATP to ADP (i.e. adenosine diphosphate) which helps release energy in cellular respiration.
​
High-performance muscles require more energy (ATP), which means more mitochondria. Because of this, evolutionary conflicts exist where mitochondria and contractile elements (fibers in muscle cells) begin to compete for cell space. Muscles that have adapted to rapid contractions generally have less tension when compared to muscles that contract at slower rates. Large concentrations of mitochondria and greater sarcoplasmic reticulum contribute to the cross-sectional reduction of contractile elements which affect tension. Isotonic contractions occur when tension in the muscle remains constant despite a change in muscle length, like when walking or running. The rate of contraction is dependent on how quickly myosin hydrolyzes ATP and produces cross-bridge actions. Force will produce at higher rates when more of the cross-sectional area is committed to the contractile elements in a muscle fiber.
​
Essentially, ATP is produced in 3 ways:
-
transfer of high-energy phosphate from creatine phosphate to ADP
-
glycolysis
-
oxidative phosphorylation ​
Nerve impulses signal each muscle fiber which allows for the ability of movement. This is accomplished from the initial thought input in the brain that send nerve signals to the muscles. In the muscle, the fibers convert the input of chemical energy (i.e. ATP) into an output of mechanical energy (i.e. muscle contraction; movement). ​
This contractile activity requires ATP hydrolysis to provide energy for cross-bridge power strokes. A power stroke occurs when a single cross-bridge cycle uses one molecule of ATP and moves the actin filament about 10 nm toward the M-line. The M-line is at cross-connecting elements and binds creatine kinase, which facilitates the reaction of ADP and phosphocreatine into ATP and creatine. Additionally, the contractile activity supports the calcium pump in the sarcoplasmic reticulum. The sarcoplasmic reticulum (SR) is a type of endoplasmic reticulum (ER) in which isolated muscle cells store calcium ions. Calcium ATPase transfers the calcium ions from the cell's intracellular fluid (ICF) to the lumen (i.e. membrane-defined space) which uses energy from ATP hydrolysis during muscle relaxation. ​
The muscle's surrounding blood vessels help supply oxygenated blood. Small rich blood vessels (i.e. capillaries) distributed within the muscle aid in delivering this oxygen. Veins within the muscle aid in circulation during contraction where blood is forced out. In order for the muscles to keep up with the high endurance, they require a sufficient intake of oxygen through panting.
contractile activity and muscular energy
skeletal muscles
Skeletal muscles are made up of two types of fibers. ​
​
Slow-twitch muscle fibers:
-
"red" muscle
-
dense with capillaries and rich in mitochondria and myoglobin (gives muscle tissue its red color)
-
carries more oxygen and sustains aerobic activity
-
endurance of this muscle is increased by aerobic training
Fast-twitch muscle fibers:
-
"white" muscle
-
tend to have fewer mitochondria
-
larger energy stores
-
can contract more quickly and with a greater force can sustain only short, anaerobic bursts of activity before muscle contraction becomes painful or fatigued become faster and stronger in response to short, intense activities.
Smooth muscles
Smooth muscles have myosin that hydrolyzes ATP slowly, resulting in slow contractions (when compared to skeletal muscles). Because of the slow rate, these contractions can be held for longer periods. They does so by utilizing available cross-bridges with bits of energy.
Contractions in smooth muscle occur when calcium ions bind to calmodulin. This binding stimulates myosin light-chain kinase (MLCK), which phosphorylates myosin. This then increases the activity of ATPase to myosin heads that establish cross-bridges to actin in order to pull them which promotes contraction. When calcium input decreases, it unbinds from calmodulin and deactivates MLCK.
​
These muscles act as a lining in organs and can be found in the eye and at the base of hairs. Automatic response to fear or even climate will cause hairs to stand up. Smooth muscle contractions are essential to maintaining homeostasis in the body through involuntary functions.
Additional Muscle Illustrations of Lycaon pictus

Figure.
Illustration of the muscles and bones of the African wild Figure 8: Medial view of the right antebrachium in L. pictus, including mm. biceps brachii and brachioradialis.
​
Illustration of the muscles and bones of the African wild dog forearm. The bone labeled "metacarpal 1" is the vestigial first digit. Muscles of the forearm are adapted to provide stability to the wrist and elbow, which assists with endurance running.
Illustrations by Brent Adrian of Midwestern University.
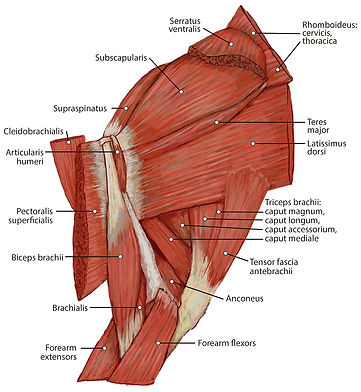
Figure
Medial view of the right shoulder and brachium in Lycaon pictus.

Figure
Palmar view of manus muscles in L. pictus (right side): (A) superficial view; (B) deep view.
Illustration of the muscles and bones of the African wild dog forearm. The bone labeled "metacarpal 1" is the vestigial first digit. Muscles of the forearm are adapted to provide stability to the wrist and elbow, which assists with endurance running.
Illustrations by Brent Adrian of Midwestern University.
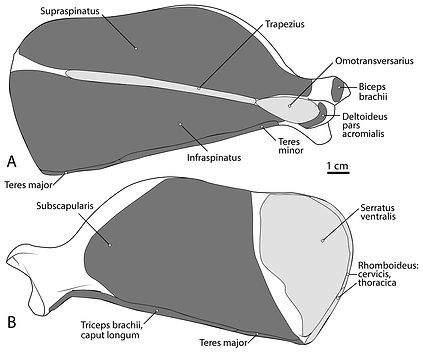
Figure.
Scapula muscle maps for L. pictus (right side): (A) lateral view, (B) medial view. Here and in subsequent figures, dark grey indicates muscle origins and light grey indicates muscle insertions.
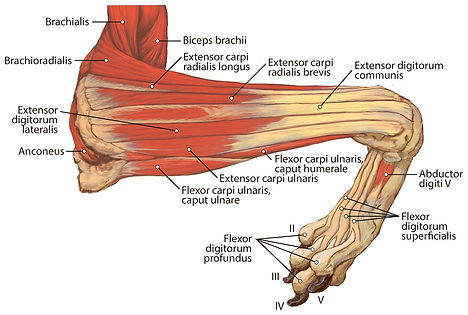
Figure.
Lateral view of the superficial right antebrachium in L. pictus, including mm. biceps brachii, brachialis, and anconeus.
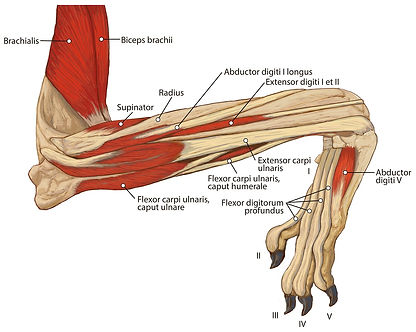
Figure.
Lateral view of the deep right antebrachium in L. pictus.

Figure.
Lateral view of the right shoulder and brachium in L. pictus.
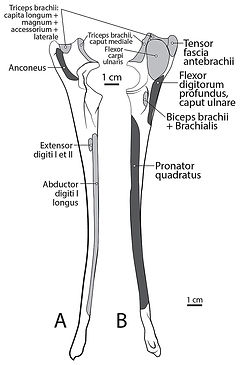
Figure.
Ulna muscle maps for L. pictus (right side): (A) lateral view; (B) medial view.
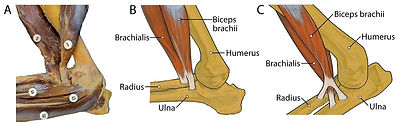
Figure.
Insertions of mm. biceps brachii and brachialis: (A) photograph of Lycaon pictus, (B) illustration of L. pictus, (C) illustration of Canis familiaris.
Numbers indicate: 3, m. biceps brachii; 9, m. brachialis; 42, m. flexor digitorum superficialis; 43, m. flexor digitorum profundus; 44, m. flexor carpi radialis. Note that in L. pictus, the tendons of mm. biceps brachii and brachialis fuse to insert together onto both the radius and ulna, while in C. familiaris the m. brachialis tendon travels through a split in the tendon of m. biceps brachii to insert exclusively onto the ulna.
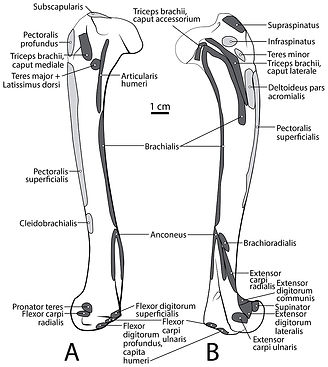
Figure.
Humerus muscle maps for L. pictus (right side): (A) medial view, (B) lateral view.
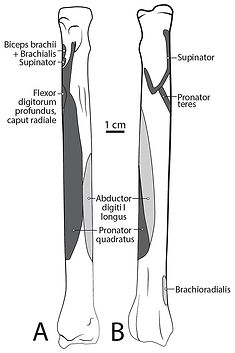
Figure.
Radius muscle maps for L. pictus (right side): (A) medial view; (B) lateral view.
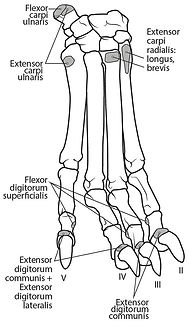
Figure.
Dorsal manus muscle maps for L. pictus (right side).
© 2021 by Brandy Turner. Proudly created with Wix.com